Prototyping is pivotal in manufacturing, providing a tangible assessment of a design’s functionality and aesthetics before full-scale production. Its significance lies in identifying design flaws, assessing user interaction, and refining products to meet technical requirements and consumer expectations. In today’s fast-paced market, rapid prototyping facilitates quicker design iterations and immediate feedback, ensuring products meet high standards and reach the market swiftly.
What is Rapid Prototyping?
Rapid prototyping represents a revolutionary approach in manufacturing, facilitating the swift creation of physical parts, models, or assemblies using 3D CAD. This innovative process predominantly employs additive manufacturing, popularly known as 3D printing.
The essence of rapid prototyping lies in its ability to quickly transform digital models into tangible prototypes. These rapid prototypes vary in fidelity: high-fidelity prototypes closely resemble the final product, while low-fidelity prototypes display more pronounced differences from the end product, often used for preliminary concept validation.
The operational mechanics of rapid prototyping encompass a spectrum of manufacturing techniques. While layered additive manufacturing is its most common form, the process is not limited to it. Other more conventional processes include high-speed machining, casting, moulding, and extruding, each serving distinct prototyping needs.
For instance, subtractive processes involve carving out materials using milling, grinding, or turning to achieve the desired shape. Compressive techniques, on the other hand, mould semi-solid or liquid materials into form before solidification, as seen in casting or compressive sintering.
These diverse processes underline the versatility of rapid prototyping, making it adaptable to various design and material requirements.
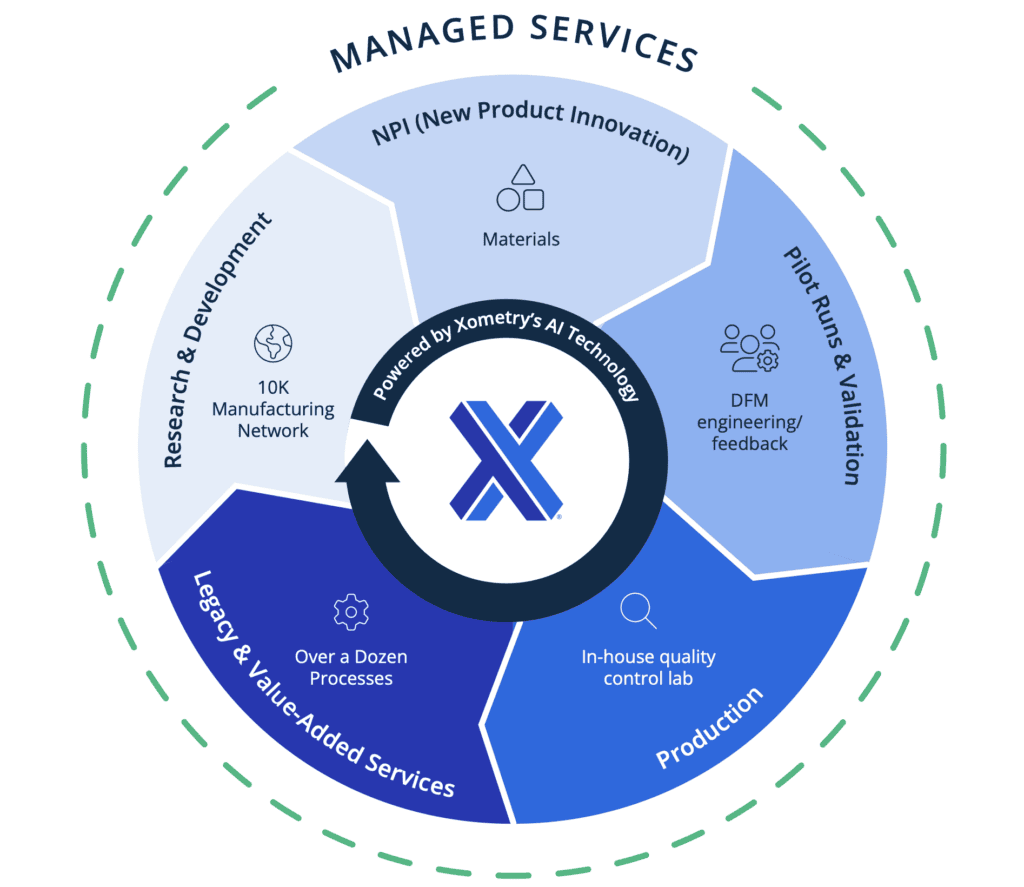
Advantages of Prototyping in Product Development
- Accelerated product development: Rapid prototyping allows for faster iteration and development cycles compared to traditional methods, reducing time-to-market for products. This rapid turnaround is essential in today’s fast-paced market environment, where the ability to swiftly move from concept to product can set a company apart.
- Cost savings: By identifying design flaws and functional issues early in the development process, prototyping helps avoid costly revisions during later stages of production.
- Enhanced creativity: Rapid prototyping encourages experimentation and innovation by providing quick feedback on design concepts, fostering a more iterative and creative approach to product development.
- Real-time feedback: Testing prototypes with real users allows for immediate feedback, enabling designers to make informed decisions and adjustments based on user preferences and needs.
- Customization and personalization: Rapid prototyping enables the creation of custom and personalized products tailored to specific user requirements, enhancing customer satisfaction and loyalty.
- Risk mitigation: By allowing for early testing and validation of concepts, rapid prototyping reduces the risk of product failure and ensures that final products meet customer expectations and market demands.
Low-Fidelity vs. High-Fidelity Prototypes: Choosing the Right Approach
Low-fidelity prototypes are the initial representations of a concept, often simplistic and quickly produced. They are used primarily for testing broader concepts, like paper sketches or cardboard mock-ups. Their main purpose is to validate the overarching idea of a product, allowing for rapid iteration and user feedback at the earliest stages of design.
On the other hand, high-fidelity prototypes closely mimic the final product’s functionality, appearance, and user interface. They are more refined and provide a realistic user experience, making them ideal for detailed testing and presentations to stakeholders.
High-fidelity prototypes are typically used in the later stages of the product development process, where detailed feedback on the product’s functionality and user interface is crucial.
Exploring Rapid Prototyping Technologies
In the following table, we highlighted the advantages, considerations and suitable applications of both additive manufacturing technologies and more traditional manufacturing methods for rapid prototyping.
Rapid Prototyping Technology | Advantages | Considerations | Suitable Applications |
Stereolithography (SLA) | • Excellent surface finish • Diverse material options |
• Slower speed • The resin may become brittle • Requires support structures |
• Low-volume production • Detailed prototypes |
Selective Laser Sintering (SLS) | • Durable parts • Suitable for complex geometries • No need for support structures • Advanced surface finish with post-processing |
• Grainy surface finish | • Functional prototypes • Complex parts |
Multi Jet Fusion (MJF) | • Rapid manufacturing • No need for support structures • Advanced surface finish with post-processing |
• Rougher surface finish than SLA | • Functional prototypes • Complex parts |
Direct Metal Laser Sintering (DMLS) | • Wide variety of metal alloys • Complex geometries • Excellent material properties |
• Slow production • High costs |
• Metal prototypes • High-performance parts |
Fused Deposition Modeling (FDM) | • Affordable prototypes • Large selection of materials (including ULTEM and ABS ESD7) |
• Moderate surface finish • Lower Z-axis strength • Limited functional testing suitability |
• Concept models • Functional prototypes |
CNC Machining | • Excellent accuracy • Diverse materials |
• High setup costs • Geometric limitations |
• High-tolerance parts • Functional testing |
Vacuum Casting | • Wide range of resins • Suitable for small to medium parts • Rapid tooling |
• Surface finish varies • Potential tolerance issues • Moulds wear out quickly |
• Pre-production parts • Functional testing |
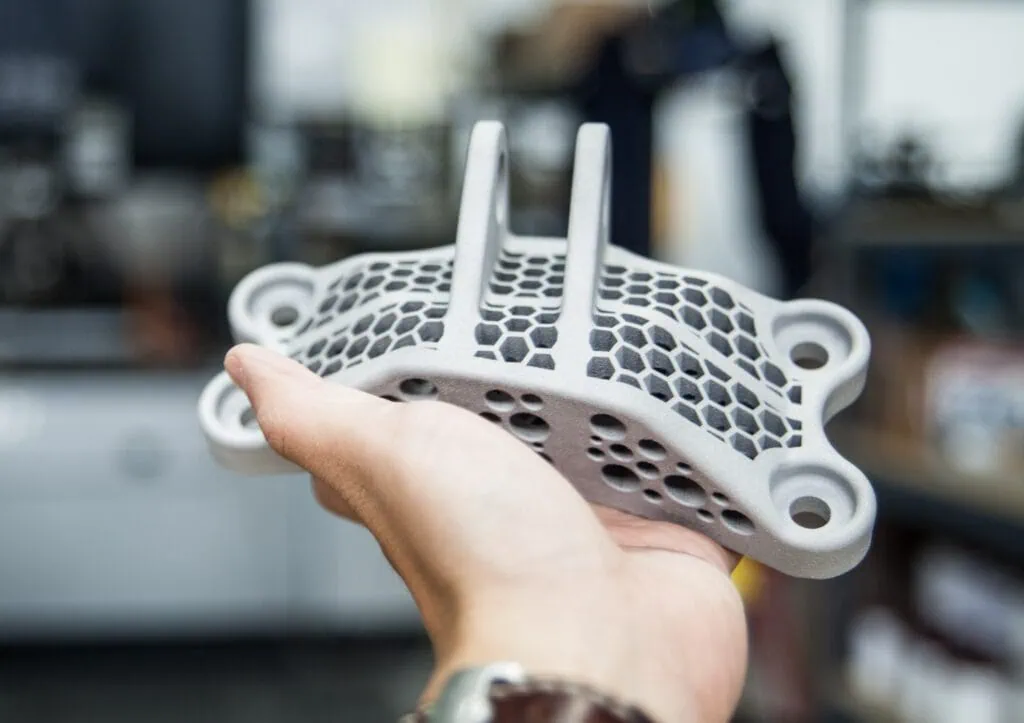
Selecting the Optimal Prototyping Technology
When selecting the optimal prototyping technology, several critical factors must be considered to ensure the successful execution of the prototyping process. The most important are as follows:
- Cost: Evaluate the initial investment, operational costs, and material expenses associated with each prototyping technology to ensure it aligns with budget constraints.
- Material compatibility: Consider the materials required for the prototype and whether the chosen technology supports the desired materials, including factors such as strength, durability, and flexibility.
- Fidelity requirements: Assess the level of detail and accuracy needed for the prototype, ensuring that the selected technology can achieve the desired fidelity, whether high or low.
- Application suitability: Determine the specific requirements of the prototype, such as functional testing, visual representation, or end-use production, and select a technology that best suits the intended application.
- Lead time: Evaluate the turnaround time for each prototyping technology, considering factors such as production speed, setup time, and lead times for materials, to meet project deadlines effectively.
- Complexity of geometry: Consider the complexity of the prototype’s geometry, including intricate features, overhangs, and internal structures, and choose a technology capable of accurately reproducing these elements.
- Scalability: Consider the scalability of the prototyping technology for future production needs, ensuring it can accommodate increased volumes and demand without compromising quality or efficiency.
Comparison of Prototyping Technologies
This table provides a concise comparison of different rapid prototyping processes against two key factors: high-fidelity prototypes and functional testing. Each rapid prototyping technique has its strengths, making them suitable for specific applications and requirements.
Rapid Prototyping Process | High-Fidelity Prototypes | Functional Testing |
Stereolithography (SLA) | ✓ Excellent surface finish and dimensional accuracy | ✓ Accurate representation of functional parts |
Selective Laser Sintering (SLS) | ✓ High level of detail and accuracy | ✓ Suitable for testing parts in end-use materials |
Multi Jet Fusion (MJF) | ✓ Excellent surface finish and properties | ✓ Capable of producing parts with consistent mechanical properties |
Direct Metal Laser Sintering (DMLS) | ✓ Produces metal parts with excellent material properties | ✓ Allows for testing parts in actual metal materials |
Fused Deposition Modeling (FDM) | ✗ Limited surface finish and dimensional accuracy | ✓ Suitable for rapid prototyping and functional testing of simple part |
CNC Machining | ✓ Excellent accuracy and dimensional precision | ✓ Capable of producing functional prototypes in various materials |
Vacuum Casting | ✓ Capable of producing high-quality, detailed prototypes | ✓ Ideal for functional testing of small to medium pre-production parts |
High-Fidelity Prototypes
- Stereolithography, selective laser sintering, multi Jet Fusion, direct metal laser sintering (also called selective laser melting), CNC machining, and vacuum casting are all capable of producing high-fidelity prototypes with excellent surface finish, dimensional accuracy, and intricate details.
- Each technology offers unique advantages such as the ability to produce parts in specific materials (SLA, SLS, DMLS), excellent material properties (DMLS), or high-quality surface finish (vacuum casting).
Functional Testing
- Stereolithography, selective laser sintering, multi jet fusion, direct metal laser sintering, CNC machining, and vacuum casting are suitable for functional testing due to their ability to produce accurate representations of functional parts.
- FDM additive manufacturing process is also suitable for functional testing, despite its limitations in surface finish and dimensional accuracy, as it can rapidly produce functional prototypes of simple parts.
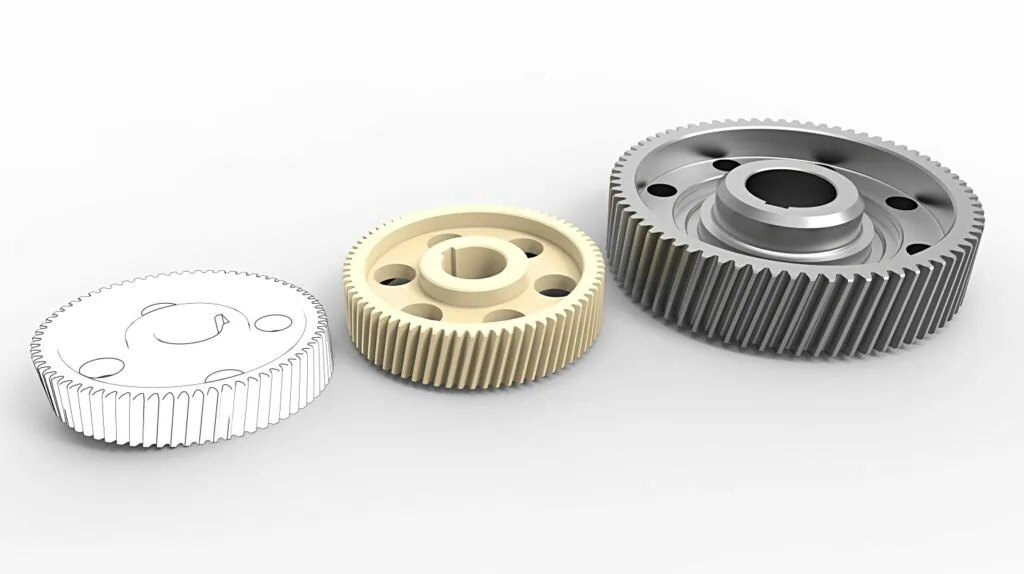
Best Practices for Effective Rapid Prototyping
- Swift development and testing: Rapid prototyping necessitates swift development to create prototypes promptly. This enables teams to test ideas quickly and gather immediate feedback from real users. By developing multiple iterations rapidly, teams can refine prototypes promptly, accelerating the overall development process and reducing time-to-market.
- Iterative improvement: Adopting rapid testing cycles allows for iterative improvements throughout the prototyping process. Real-world feedback from users is invaluable for identifying areas of improvement, enabling designers to refine custom prototypes and enhance usability. Iterative improvement ensures that prototypes evolve dynamically, resulting in more refined and user-friendly end products.
- Stakeholder involvement: Involving stakeholders, including customers, team members, and investors, is crucial for successful rapid prototyping. Regular engagement ensures alignment with business objectives and user needs, guiding the development process in the right direction.
- Continuous learning: Embracing a culture of continuous learning and adaptation is vital for ongoing improvement in rapid prototyping. Using insights gained from prototyping activities to inform future development iterations fosters innovation and growth. By continuously learning from experiences and adapting strategies accordingly, teams can refine their approach to prototyping and drive continuous improvement.
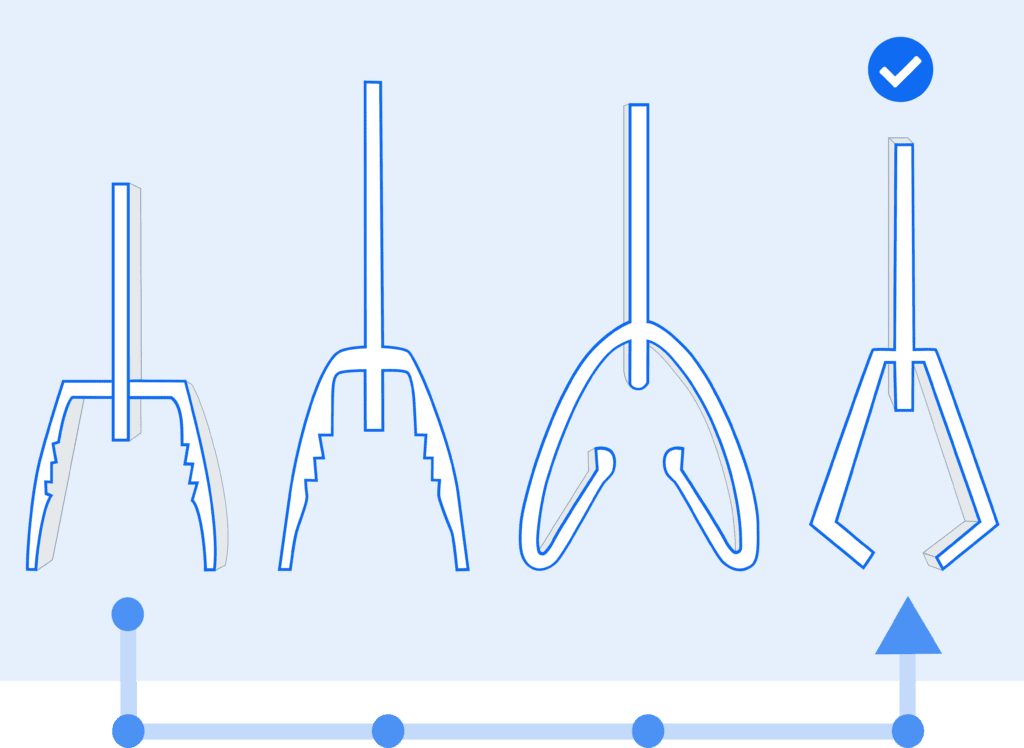
The Evolution and Impact of Rapid Prototyping in Manufacturing
Rapid prototyping stands as a cornerstone in modern manufacturing, revolutionizing the product development process. By significantly enhancing efficiency, creativity, and precision, rapid prototyping accelerates design iterations, enables iterative improvement, and facilitates cost-effective testing with real users. The diverse range of rapid prototyping technologies, from 3D printing to more traditional manufacturing processes like CNC machining, offers tailored solutions for various industry needs, bridging the gap between conceptual design and final product realization.
As rapid prototyping techniques continue to evolve with advancements in materials, precision, and speed, they are poised to further revolutionize product development, opening new frontiers in manufacturing. Ultimately, rapid prototyping signifies a transformative era in how products are designed, tested, and brought to market, shaping the future of manufacturing.